ProtonBeamIntro: Difference between revisions
(Created page with "==Introduction== The first in the chain of the Mu2e magnets is the Production Solenoid (PS), shown in Figure 1. The role of PS is to collect and focus pions and muons generat...") |
No edit summary |
||
(6 intermediate revisions by 2 users not shown) | |||
Line 1: | Line 1: | ||
==Introduction== | ==Introduction== | ||
The | Mu2e requires a high intensity, pulsed proton beam to produce large numbers muons. The experiment relies on Fermilab’s Booster, which is also used by other Fermilab experiments, such as g-2, to provide the protons. The beam for Mu2e can be delivered while other experiments are running simultaneously. While the beam of protons must be intense during these pulses in order to deliver enough muons to the stopping target for Mu2e to reach its design sensitivity, the beam of protons must also disappear between pulses. This allows for the muons captured by the experiment to decay over time, and these decay products to be measured. Protons delivered between pulses will contribute to the experiment’s backgrounds, and their rate will be measured with the extinction monitor. | ||
[[File: | [[File:ProtonBeamlineArial.jpg|thumb|center|500px|Figure 1. Layout of the Mu2e facility (lower right) relative to the accelerator complex that provides proton beam to the detector. Protons are transported from the Booster through the MI-8 beamline to the Recycler Ring where they will circulate while they are re-bunched by a 2.5 MHz RF system. The reformatted bunches are kicked into the P1 line and transported to the Delivery Ring where they are slow extracted to the Mu2e detector through a new external beamline. ]] | ||
==General Requirements== | |||
Protons designated for Mu2e are acquired from the Booster during the available portions of the Main Injector timeline when slip-stacking operations are underway for NOνA. Two Booster proton batches, each containing 4.0 × 10<sup>12</sup> protons with a kinetic energy of 8 GeV, are extracted into the MI-8 beamline and injected into the Recycler Ring. After each injection, the beam circulates for 90 msec while a 2.5 MHz bunch formation RF sequence is performed. This RF manipulation coalesces each proton batch into four 2.5 MHz bunches occupying one seventh of the circumference of the Recycler Ring. Each of these bunches will be synchronously transferred, one at-a-time, through existing transfer lines to the Delivery Ring, where the beam is held in a 2.4 MHz RF bucket during resonant extraction to the experiment through a new external beamline. To help control the spill rate uniformity during resonant extraction a technique known as RF knockout will be used. RF knockout will allow for fast transverse heating of the beam. It will also serve as a feedback tool for fine control of the spill rate. The resonant extraction process will not completely remove the entire beam, so what remains must be disposed of in a controlled way. Therefore, a beam abort system will be required for the Delivery Ring to “clean up” beam that remains after resonant extraction is complete. The resonant extraction system will inject ~ 3 × 10<sup>7</sup> protons into the external beamline every 1.7 µs (the revolution period of the Delivery Ring). An extinction system, in the form of a high frequency AC dipole , is required to suppress unwanted beam between successive pulses that can generate experimental backgrounds. After transiting the extinction system the proton pulses are delivered to the production target located in the evacuated warm bore of a high-field superconducting solenoid. The proton beam will have a transverse radius of about 1 mm (rms) and will be about 250 ns in duration. The proton beam deflects in the magnetic field of the solenoid before striking the production target, complicating the final focus beamline optics and steering. The production target is a radiatively cooled tungsten rod about the size and shape of a pencil. Not all of the proton beam interacts in the production target. The unspent beam is absorbed in an air-cooled beam absorber downstream of the production target. A monitor, located above the beam absorber, will measure scattered protons as a function of time to provide a statistical measure of the residual beam between pulses that traverses the extinction system. The proton delivery scheme is shown in Figure 1. The Mu2e proton beam requirements are described in [1]. | |||
Most of the infrastructure required to deliver proton beam to the Mu2e production target already exists or will exist before Mu2e needs it. The g-2 experiment, scheduled to take data before Mu2e, requires much of the same infrastructure. To satisfy the common needs of both projects a program to develop a Muon Campus through a series of Accelerator Improvement Plans (AIP) and General Plant Projects (GPP) has been initiated. The accelerator infrastructure required exclusively by Mu2e is part of the Mu2e Project and includes: | |||
*Resonant Extraction System | |||
*MHz Delivery Ring RF system | |||
*Mu2e external beamline • Extinction System | |||
*Extinction Monitor System • Production Target | |||
*Radiation Safety and Shielding | |||
*Beamline instrumentation and controls | |||
*Diagnostic Beam Absorber | |||
*Proton Target Beam Absorber. | |||
These elements are described in detail in this Technical Design Report[2]. | |||
[1] R. Bernstein et al., “Mu2e Proton Beam Requirements,” [http://mu2e-docdb.fnal.gov/cgi-bin/ShowDocument?docid=1105 Mu2e-doc-1105].<br> | |||
[2] Mu2e Mu2e Technical Design Report [http://mu2e-docdb.fnal.gov/cgi-bin/ShowDocument?docid=4299 Mu2e-doc-4299]. | |||
[[Category:Experiment]] | |||
[[Category:Tutorial]] | |||
[ | |||
Latest revision as of 01:58, 23 September 2022
Introduction
Mu2e requires a high intensity, pulsed proton beam to produce large numbers muons. The experiment relies on Fermilab’s Booster, which is also used by other Fermilab experiments, such as g-2, to provide the protons. The beam for Mu2e can be delivered while other experiments are running simultaneously. While the beam of protons must be intense during these pulses in order to deliver enough muons to the stopping target for Mu2e to reach its design sensitivity, the beam of protons must also disappear between pulses. This allows for the muons captured by the experiment to decay over time, and these decay products to be measured. Protons delivered between pulses will contribute to the experiment’s backgrounds, and their rate will be measured with the extinction monitor.
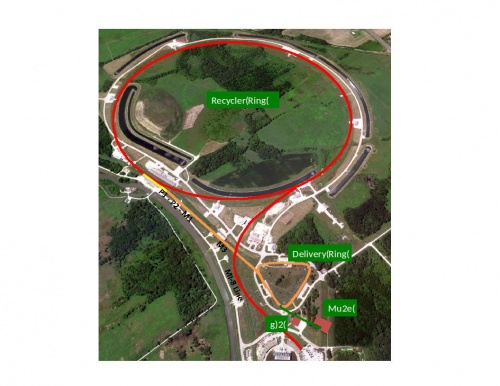
General Requirements
Protons designated for Mu2e are acquired from the Booster during the available portions of the Main Injector timeline when slip-stacking operations are underway for NOνA. Two Booster proton batches, each containing 4.0 × 1012 protons with a kinetic energy of 8 GeV, are extracted into the MI-8 beamline and injected into the Recycler Ring. After each injection, the beam circulates for 90 msec while a 2.5 MHz bunch formation RF sequence is performed. This RF manipulation coalesces each proton batch into four 2.5 MHz bunches occupying one seventh of the circumference of the Recycler Ring. Each of these bunches will be synchronously transferred, one at-a-time, through existing transfer lines to the Delivery Ring, where the beam is held in a 2.4 MHz RF bucket during resonant extraction to the experiment through a new external beamline. To help control the spill rate uniformity during resonant extraction a technique known as RF knockout will be used. RF knockout will allow for fast transverse heating of the beam. It will also serve as a feedback tool for fine control of the spill rate. The resonant extraction process will not completely remove the entire beam, so what remains must be disposed of in a controlled way. Therefore, a beam abort system will be required for the Delivery Ring to “clean up” beam that remains after resonant extraction is complete. The resonant extraction system will inject ~ 3 × 107 protons into the external beamline every 1.7 µs (the revolution period of the Delivery Ring). An extinction system, in the form of a high frequency AC dipole , is required to suppress unwanted beam between successive pulses that can generate experimental backgrounds. After transiting the extinction system the proton pulses are delivered to the production target located in the evacuated warm bore of a high-field superconducting solenoid. The proton beam will have a transverse radius of about 1 mm (rms) and will be about 250 ns in duration. The proton beam deflects in the magnetic field of the solenoid before striking the production target, complicating the final focus beamline optics and steering. The production target is a radiatively cooled tungsten rod about the size and shape of a pencil. Not all of the proton beam interacts in the production target. The unspent beam is absorbed in an air-cooled beam absorber downstream of the production target. A monitor, located above the beam absorber, will measure scattered protons as a function of time to provide a statistical measure of the residual beam between pulses that traverses the extinction system. The proton delivery scheme is shown in Figure 1. The Mu2e proton beam requirements are described in [1].
Most of the infrastructure required to deliver proton beam to the Mu2e production target already exists or will exist before Mu2e needs it. The g-2 experiment, scheduled to take data before Mu2e, requires much of the same infrastructure. To satisfy the common needs of both projects a program to develop a Muon Campus through a series of Accelerator Improvement Plans (AIP) and General Plant Projects (GPP) has been initiated. The accelerator infrastructure required exclusively by Mu2e is part of the Mu2e Project and includes:
- Resonant Extraction System
- MHz Delivery Ring RF system
- Mu2e external beamline • Extinction System
- Extinction Monitor System • Production Target
- Radiation Safety and Shielding
- Beamline instrumentation and controls
- Diagnostic Beam Absorber
- Proton Target Beam Absorber.
These elements are described in detail in this Technical Design Report[2].
[1] R. Bernstein et al., “Mu2e Proton Beam Requirements,” Mu2e-doc-1105.
[2] Mu2e Mu2e Technical Design Report Mu2e-doc-4299.