StoppingTargetMonitorIntro: Difference between revisions
(Created page with " ==Introduction== Given the complexity of generating and collecting low energy negative muons in the production solenoid, and transporting them via the transport solenoids to...") |
No edit summary |
||
Line 39: | Line 39: | ||
[[File:StmGeometry1.png|thumb|center|600px|]] | [[File:StmGeometry1.png|thumb|center|600px|]] | ||
Radioactive sources, such as Europium-152, are required for calibration purposes. The calibration and absolute efficiency of the Ge detector will be determined following ANSI N42.14-1999 “American National Standard for Calibration and Use of Germanium Spectrometers for the Measurement of Gamma-Ray Emission Rates of Radionuclides”. We expect a few percent measurement based on standard practice, well within the 10% requirement. | |||
[1] J. Miller and R. Bernstein, “Requirements & Specifications WBS 5.6 --- Muon Stopping Target Monitor,” [http://mu2e-docdb.fnal.gov:8080/cgi-bin/ShowDocument?docid=1438 Mu2e-doc-1438] <br> | [1] J. Miller and R. Bernstein, “Requirements & Specifications WBS 5.6 --- Muon Stopping Target Monitor,” [http://mu2e-docdb.fnal.gov:8080/cgi-bin/ShowDocument?docid=1438 Mu2e-doc-1438] <br> |
Revision as of 15:42, 17 November 2017
Introduction
Given the complexity of generating and collecting low energy negative muons in the production solenoid, and transporting them via the transport solenoids to the target in the detector solenoid, it is evident that some means of confirming the rate and integral number of negative muons that stop in the muon-stopping target is necessary. It is equally evident that such a device should prove to be useful in the initial process of tuning conditions for the proton beam and the solenoids. Requirements
The goal is to determine the number of stopped muons to an accuracy of 10% (at 1 standard deviation) over the course of the experiment [1].
It is possible to imagine a “dead-reckoning” calculation based on a simulation of the beamline, modeling of the stopping target, etc. However, it seems prudent to directly monitor some process that signals the capture of a muon on an aluminum nucleus.
One could monitor the X-rays emitted during the formation of a muonic atom and the associated cascade to the 1s state. The highest yield X-ray is the 2p →1s radiative transition that confirms the arrival of a muon in the ground state. Other observable X-rays having substantial yields and signaling arrival in the 1s state, are the 3p→1s, and the 4p→1s. Typically the 3d→2p transition that populates the 2p state also appears in the energy spectrum. In addition, muons that stop in impurities in the target, such as oxygen, generate X-rays with their own characteristic energies, potentially allowing us to determine the fraction of muons that stop in impurity nuclei.
A solid-state detector such as Ge is typically used for detecting such X-rays. However, these detectors have a relatively slow time response and are not normally used in an environment as intense as expected at Mu2e. The Mu2e beam has an intense electron component at early times (beam flash); the same aluminum that stops muons will then produce bremsstrahlung photons (from those electrons) at a rate we calculate to be 51 MHz/cm2 with a mean energy of 1.4 MeV. The interesting muons arrive about 100 nsec after the flash and produce their X-rays within picoseconds of their arrival. Commercial off-the-shelf detectors and their electronics cannot manage these rates (MeV/sec limits) and their associated electronics are not fast enough [2]. This solution is still under study as of this writing but is not the baseline. The detector would also experience radiation damage from the beam flash and the neutron flux is problematic, requiring annealing once per day or less.
To eliminate the rate and radiation issues created by the flash, the baseline approach is to detect delayed gamma rays from the decays of radioactive nuclei produced in the muon capture process, rather than muonic X-rays. These delayed gammas, like the X-rays, are unique to the target material, and information on their energies and intensities is available in the literature. For 27Al, a 27Mg nucleus is produced in 13% of muon captures. The excited 27Mg beta-decays back to an excited state of 27Al with a half-life of 9.5 minutes. This 9.5 minute half-life gives us an opportunity to eliminate the problems of the beam flash. The proton beam structure of Mu2e will be a stream of proton pulses for 0.5 s followed by 0.8 s idle. The gamma spectrum would be acquired during the relatively background-free idle period and the beam would be blocked using a thick collimator during the time the beam is on, eliminating the problems created by the beam flash [3]. After the beta emission, the excited 27Al returns rapidly to the ground state, producing an 844 keV gamma ray 72% of the time.
Three requirements determine the best location for the Ge detector to view the muonstopping target:
- The detector should only view the target, if possible. Hence the first requirement is for good collimation ahead of the detector.
- Because of the extraordinarily high X-ray and gamma rates the detector must be far from the source, along a low attenuation path for photons.
- The detector must lie beyond the Detector Solenoid (DS) magnetic field where it can be serviced periodically and annealed to repair radiation damage.
The Ge detector performance must be such that annealing to recover from radiation damage is not required more often than once per 2 months of calendar time. Technical Design
A preliminary spectrum of delayed gammas from muon capture in an aluminum target measured with a germanium detector is shown in Figure 1. The gamma ray can be detected with a high resolution photon detector. The gamma ray is unique to the target, and no other material in view of the detector will consist of aluminum. Measurements by the AlCap experiment [4] will establish the normalization between the number of stopped muons and the rate of 844 keV gammas. Good energy resolution is desirable in order to deliver good signal to noise and to resolve the 844 keV peak from gamma rays with nearby energies. Commercially available intrinsic germanium detectors will be used because they deliver excellent resolution (~2 keV@1.33 MeV) together with high efficiency.
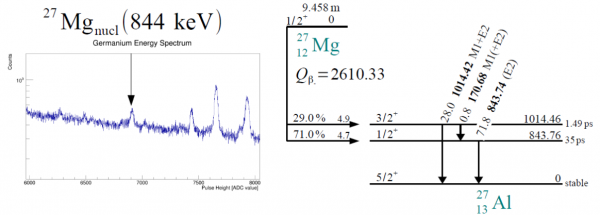
Figure 2 shows a preliminary plot of the singles spectrum (self-triggered spectrum) from the AlCap experiment. Muons are stopped at the estimated rates of 3 kHz in Aluminum and 4 kHz in lead shielding and other materials. Despite the large number of stops in materials other than aluminum and the absence of any cuts on the gamma time relative to the muon stopping time, the 347 keV gamma line is clearly visible above the background. In the case of Mu2e, the live window will be delayed in time from the stopped muons, therefore we expect the signal to noise to improve. This will be quantified in the future in the AlCap data by vetoing any Ge data within a few muon lifetimes after the muon’s arrival. Care will be taken in Mu2e to avoid viewing materials that are activated or stopping locations other than the target, therefore we expect that the background situation will be improved relative to that at AlCap.
Germanium is moderately susceptible to radiation damage from neutrons and ionizing radiation (photons, charged particles). Most of the damage is readily annealed by heating the Ge for about a day. To provide continuous monitoring, multiple detectors would have to available to be rotated into the beam and annealed very often, which is undesirable. In order to circumvent this, we propose to take advantage of the beam cycle and delayed nature of the gamma rays to block most of the harmful radiation from impinging upon the Ge detector by employing a blocking shutter. The primary proton beam arrives in a stream of 260 ns wide pulses separated by 1695 ns, for a period of 0.5 s, followed by 0.8 seconds during which there is no beam. A shutter will block the beam for the 0.5 s when the proton pulses arrive, but will allow transmission during the 0.8 second beam-off period. For a 5 cm diameter active area in the detector, positioned 15 m downstream from the target, the fractional geometric acceptance is 1x10-6 . With a muon stopping rate of 1x1010 Hz, the incident rate of 844 keV gammas is 480 Hz.
In the Mu2e experiment, the Ge detector will be located near the axis of the Detector Solenoid, downstream of the bore vacuum, muon beam stop and endcap shielding. The detector is enclosed in a small concrete house to provide shielding (see Figure 3). It will view the stopping target through a 10 cm diameter, 3 m long stainless steel pipe connected to the center of the downstream end of the Detector Solenoid. Collimators inside the pipe will allow full view of the stopping target while limiting the view of other materials such as the last collimator in the TS solenoid (TS5). A dipole magnet will sweep away electrons in the beam. The beamline will include a shutter that will be triggered to move into the beamline during the beam, and move out to provide a clear aperture between beam spills. A vacuum window will separate the Detector Solenoid from the collimation pipe end window.
Radioactive sources, such as Europium-152, are required for calibration purposes. The calibration and absolute efficiency of the Ge detector will be determined following ANSI N42.14-1999 “American National Standard for Calibration and Use of Germanium Spectrometers for the Measurement of Gamma-Ray Emission Rates of Radionuclides”. We expect a few percent measurement based on standard practice, well within the 10% requirement.
[1] J. Miller and R. Bernstein, “Requirements & Specifications WBS 5.6 --- Muon Stopping Target Monitor,” Mu2e-doc-1438
[2] R. Bernstein, “Stopping Target Monitor Rates,” Mu2e-doc-4010
[3] R. Bernstein, “Mu2e Muon Stopping Target Monitor Design Considerations,” Mu2e-doc-4131
[4] J. Quirk and J. Miller, “AlCap Update”, Mu2e-doc-4057
AlCap experiment